Colliding Galaxy Clusters May Hold Clues To Dark Matter
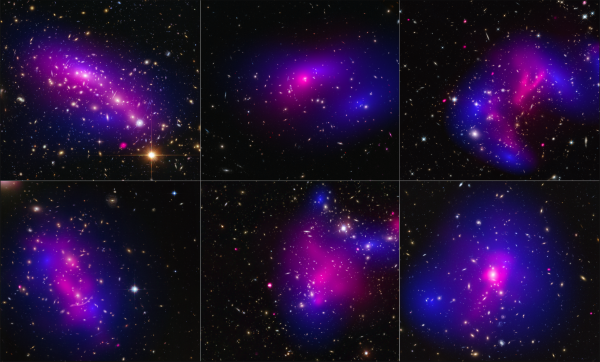
Sound is carried by periodic vibrations of atoms in gases, liquids and solids. When we talk to each other, the vocal chords of the speaker vibrate, causing the air coming from his lungs to vibrate as well. This creates sound waves, which then propagate through the air until they hit a listener’s eardrums and make them vibrate as well. From these vibrations, the listener can then reconstruct the speaker’s words.
Sound is affected by the surroundings in which it travels and by the frequency of the sound waves. We design musical instruments to manipulate the sound waves they produce. Further, we know that there are sound waves that are outside the range of human hearing, such as those produced by a dog whistle. As physicists have researched sound both inside and outside the range of human hearing, interesting properties have been discovered.
More than a hundred years ago, physicists understood that heat is simply the energy stored in the vibrations of atoms, and therefore realized that heat and sound are related. Now my lab showed experimentally for the first time that these atomic vibrations have magnetic properties too.
In the 1930s, physicists started modeling atomic vibrations as particles. This is similar to the concept of light as both a wave and a particle we call a photon. Physicists called the sound wave particles “phonons,” derived from the Greek word for sound.
Today, physicists treat phonons as quasi-particles, having both wave and particle properties. Phonons carry both sound and heat. In metals, heat is carried primarily by the movement of electrons in the atoms. However, in all other materials, heat is carried almost exclusively by the phonons.
So the mechanical, acoustic and thermal properties of sound waves have long been established. Yet, before now, nobody ever imagined that sound waves might also have magnetic properties.
Kevin Fitzsimons, The Ohio State University, CC BY-NC-ND
In the March 23 issue of Nature Materials, we offer experimental proof that sound waves do interact with external magnetic fields.
The experiment was carried out on a large, single crystal of a very pure semiconductor, indium antimonide, which had been cut into two unequal sections and then cooled to about -445F (-265C). A controlled amount of heat was made to flow in each section separately. At these temperatures, the phonons can be thought of as individual particles, like runners on a racetrack each carrying a little bucket of heat.
Renee Ripley, The Ohio State
University, CC BY-NC-ND
In the small section, the phonons often run into the walls, which slows them down. The small section is used as a reference, to make the experiment independent of the other properties of the solid that might interfere. In the large section, the phonons can go faster, and they don’t run into the walls as much as into each other. When we apply a magnetic field, they tend to run into each other more frequently. Because the magnetic field increases the number of collisions, it also slows the phonons down and lowers the amount of heat they carry by 12%.
We think this is due to the electrons that rotate in orbits around each atom in the solid. The orbital motion of these electrons emits a very small intrinsic magnetic field that interacts with the externally applied field – an effect called “diamagnetism.“ This property exists even in substances we don’t traditionally think of as magnetic, such as glass, stone or plastic. When the atoms vibrate due to the passing of the phonons, this interaction creates a force on the atoms that makes the phonons collide with each other more often.
At this point, we’ve just described a new concept, something that had never been thought of before. Engineers can perhaps use this concept to control heat and sound waves magnetically. Sound waves can be effectively steered already by using multiple sources of sound, as is done in ultrasound imaging systems, but controlling heat conduction is much harder.
Conversion of heat into electrical or mechanical power, as is done in engines and in power stations, supplies over 90% of the energy humanity uses. Therefore, being able to control heat conduction at will could have an enormous impact on energy production, though, obviously, applications of this emergent concept are still quite a way in the future.
This article was originally published on The Conversation.
Read the original article.
A solar eclipse is a rare event to witness first hand. A wag might add that once you’ve factored in the British weather they’re rarer still, however observers in some areas of the UK managed to peer through the clouds and experience a partial solar eclipse from Exeter and Truro in the South-West to Nottingham in the Midlands, and Hull and Newcastle and the North-East.
The reason solar eclipses are rare is due to the orbit of the moon around the Earth, which is inclined by about five degrees to Earth’s orbit around the sun. This means that not only must the moon be a new moon in order to put it in the sky during daylight hours, but it must also be in just the correct arc of its orbit that it is aligned directly between the Earth and the sun.
A lunar eclipse, where the Earth comes between the sun and the moon, darkening the moon by blocking the sun’s light, can be seen by most people on the dark side of the Earth. But not everyone on the daylight side of the Earth will see a solar eclipse, because the shadow of the moon on the Earth’s surface covers only a band across the planet. To witness complete totality during an eclipse observers must be in an even more narrow band just 250km (150 miles) wide. This is why the best views were restricted to the islands of Svalbard and the Faroes this year.
Scientifically speaking, eclipses have had a rich history in helping scientists conduct certain types of experiment and making unique discoveries.
A total solar eclipse was used to test gravity as described by Newtonian physics against predictions made by Einstein’s new theory of General Relativity. Both forms of gravitation predict that light can be bent around the sun, but by different amounts. In order to see other celestial objects – such as background stars – near the disk of the sun, the only thing to do was await a total solar eclipse. This happened in 1919, when Arthur Eddington and others simultaneously made measurements of the positions of stars close to the sun during an eclipse. They found that light was bent by the extent predicted by general relativity, rather than classical Newtonian physics. This proved to be the first of many spectacular successes for general relativity.
The sun is not the only celestial body to be eclipsed – or occulted – by the moon. In 1962, a lunar occultation technique was employed at the Parkes Telescope to determine that a quasar (a bright point-like object that appeared like a star) consisted of two elements. This use of occultations yielded the positions and further details of these elements which led to an astronomical revolution. Quasars are exceptionally powerful extra-galactic objects.
Today we can use the idea of occultations even to predict the weather. This uses Global Positioning Satellites (GPS) that constantly transmit their positions to listening stations worldwide. All that is required is for part of the atmosphere to occult and block the signal between one satellite and another. This results in refraction, where the radio signal is bent through the atmosphere. Critically, the amount of refraction is highly dependant upon the conditions in the atmosphere, for example water vapour density and ambient temperature. So based on these factors we can produce an instantaneous mapping of the current weather, and additional data to predict the future weather, based on the degree of refraction encountered.
Further away from home, we can use the idea of occultations to help derive the size of other planets orbiting distant stars. When a planet passes in front of a distant star in the line of sight to Earth, it creates an apparent partial eclipse that causes a dip in the observed light from that star. We can deduce the size of the planet by measuring how much light is blocked out during its transit.
So while solar eclipses are not a common sight, they’re certainly an opportunity for scientists to learn more about the world and the laws that govern the Universe – naturally occurring phenomena that we’re lucky to have the opportunity to observe from time to time.
This article was originally published on The Conversation.
Read the original article.
A total solar eclipse is an extraordinary visual and emotional experience, from the moon’s first bite out of the sun’s unblemished disc, to the moment that the daylight sky becomes dark and the sun’s blazing light is muted. The temperature drops, the moon’s shadow can be seen speeding across the Earth’s surface, and there is a sudden silence as animals settle down to sleep under the impression that night has fallen.
Yet the fact that we see a solar eclipse at all is something of a miracle. Ours is the only planet in the solar system whose moon appears from the surface to have the same angular size in the sky as that of the sun: it is 400 times smaller, but also 400 times closer. This cosmological coincidence allows the moon to completely block out the sun to reveal the ethereal beauty of its tenuous outer atmosphere, the corona, to those fortunate enough to be standing in the shadow of the moon.
John Couch Adams, who discovered Neptune, wrote in 1851:
The appearance of the corona, shining with a cold unearthly light, made an impression on my mind which can never be effaced, and an involuntary feeling of loneliness and disquietude came upon me.
I consider myself extremely fortunate to have witnessed four total solar eclipses so far. This, my fifth, will be the first solar eclipse visible in Europe since 1999, and the last until 2026. A total solar eclipse happens somewhere on the planet on average every 18 months, but for the moon’s shadow to pass over inhabited land is far less common.
Fred Espenak/NASA, CC BY
The solar eclipse will begin in the mid-Atlantic at sunrise, travelling north-east between Iceland and the UK and Ireland, before ending at the North Pole. The only islands from which totality – the moment when the moon entirely eclipses the sun leaving only a fiery halo – will be visible is remote Svalbard in the Arctic and the Danish-owned Faroe Islands, where I intend to be.
In the UK and Ireland, depending on your location, the maximum coverage will be a partial eclipse of between 85-97% of the sun’s disk, with the degree increasing towards the north. However, even almost entirely occluded in this way, the sun is still bright enough to cause severe eye damage and so precautions must be taken. A partial eclipse is best viewed through special eclipse glasses (not sunglasses), or by projecting the sun’s image onto a piece of white card using a pinhole projector – do not look directly at the sun.
Erik Christensen, CC BY-SA
Aside from being the most awe-inspiring sight in nature, solar eclipses also have immense scientific value. The element helium was discovered as a result of solar eclipse observations, decades before it was detected on Earth. Einstein’s theory of general relativity was proven correct thanks to a solar eclipse, when a star’s apparent position in the sky was “shifted” due to the gravitational influence of the sun. And the technique of spectroscopy led to the discovery that the sun’s corona holds a temperature of over 1,000,000°C – a thousand times hotter than its surface. This has become known as the coronal heating problem, something scientists are still grappling with today. Even now in the 21st century, with advanced scientific instruments both on the ground and in orbit, we cannot reproduce the conditions that nature provides during totality.
NASA, CC BY
The technology we have come to depend upon is at the mercy of catastrophic explosions that originate in the sun’s atmosphere. Solar flares and coronal mass ejections are a consequence of the sun’s contorted coronal magnetic field which, when stretched beyond breaking point, spews plumes of radiation and charged particles in the direction of Earth, putting our electronic infrastructure at risk. Studying the inner corona during a solar eclipse allows us to quantify the state of the sun’s magnetic field, which will help us understand how solar storms get accelerated and the origins of the solar wind, among other things.
It’s a bit late to start planning a North Atlantic trip now, but bear in mind that in August 2017 the moon’s shadow will traverse the entire continental US, from Oregon to the Carolinas, making it one of the most accessible total eclipses of a generation. If you possess any sense of wonder and adventure, then you owe it to yourself to find a way of experiencing this fascinating phenomenon first hand. Then you can join in the chorus heard after the moon’s shadow has passed: “When is the next one?”
This article was originally published on The Conversation.
Read the original article.
When Captain Cook sailed into Botany Bay in 1770, we did not know how many planets were in our solar system. We only knew about Mercury, Venus, Earth, Mars, Jupiter and Saturn.
Based on the orbits of these planets, 18th century European astronomers invented what is now called the Titius-Bode relation. It’s a simple empirical relation that describes the relative distances between the planets and the sun. It predicted the orbit of another planet beyond Saturn and another planet in the gap between Mars and Jupiter.
In 1781, William Herschel found Uranus – without relying on the Titius-Bode relation – but he found it in the orbit beyond Saturn where the Titius-Bode relation said it would be.
After this success, astronomers started looking for a planet between Mars and Jupiter in the orbit predicted by the Titus-Bode relation.
In 1801, Giuseppi Piazzi found a planet in the predicted position and called it Ceres. The Titius-Bode relation was on a roll.
But when Neptune was found in 1846 it wasn’t exactly where the Titus-Bode relation predicted it would be. And over the years so many small bodies have been found in orbits between Mars and Jupiter that Ceres was plutoed – demoted to an “asteroid”.
And so the Titus-Bode relation lost its shine. And like an old horse, it was put out to pasture. It was only taken seriously by numerologists and cranks.
But then along came NASA’s Kepler Space Telescope. Over the past few years Kepler has been able to detect thousands of exoplanets and hundreds of multi-exoplanet systems.
Along with my PhD student Tim Bovaird and Master’s student Steffen Jacobsen, we reasoned that if the TB relation had been such a useful (if somewhat imperfect) guide for predicting planets in our solar system, maybe it would be useful in predicting planets in the new exoplanetary systems detected by Kepler.
We checked the hundred or so systems where Kepler had found at least a few planets and we found that the majority of these exoplanetary systems adhered to the Titus-Bode relation even somewhat better than our solar system did.
Thus, we became convinced that the horse still had some miles left in her – that the semi-taboo Titus-Bode relation could provide useful hints about the periods of as-yet-undetected planets around other stars.
Last year we used a generalised Titus-Bode relation to analyse 68 multi-planet systems with four or more detected exoplanets. We made predictions for the existence of more planets in these systems, based on the Titus-Bode relation.
So far, 5% of our predictions have been confirmed. This may sound like a small percentage, but given the inability of the Kepler telescope to see Earth-sized planets or smaller, a 5% detection rate is what you would expect to see if all the predictions were true.
Aditya Chopra, ANU, adapted from NASA/JPL
Almost all of the exoplanets detected by Kepler are larger than Earth and very close to their host stars. This is almost certainly a selection bias.
It is very difficult for the Kepler telescope to spot planets that are far enough away from their host stars to be in the habitable zone (where the temperatures are in the range where H2O will be liquid water).
Using the Titus-Bode relation is a controversial indirect technique, but I think it’s the best one we have if we are interested in answering the question: How many planets (on average) are in the habitable zones of stars?
Our answer to this question is 2 ± 1 and was published this week in the Monthly Notices of the Royal Astronomical Society. The figures (above and below) illustrate our result.
Author provided
With about 300 billion stars in our galaxy, our result means there are 600 ± 300 billion planets in circumstellar habitable zones in our galaxy.
In the observable universe there are about 100 billion galaxies. Thus there are approximately 1022 stars in the observable universe and twice that many planets in circumstellar habitable zones in the universe.
That’s a lot of real estate for alien development. Not all of these habitable zone planets will be wet and rocky like the Earth, but a fair fraction (about 30%) should be. Now we need some zippy interstellar spaceships to colonise and over-populate all these worlds before the aliens do.
This article was originally published on The Conversation.
Read the original article.